Peter J. Peters (J.P.J.)
Division for Nanobiology
Center for Imaging of Cellular Nanomachines
Visualizing macromolecular organization of unperturbed cellular environments
Visualizing Nanomachines in a Cellular Context
The cellular nanocosmos is composed of numerous types of macromolecular complexes, or biological nanomachines. These form functional modules that are organized into complex subcellular networks. To date, information on the ultra-structure of these nanomachines has largely been obtained by analyzing isolated structures, using imaging techniques such as X-ray crystallography, NMR, or single particle electron microscopy (EM). It is imperative, however, to image biological complexes in their native state and within a cellular environment in order to better understand their functions in both their normal and diseased states.
In recent years, the rapid development of imaging methods has revolutionized our ability to directly visualize protein complexes at work in organelles of cells that make up tissues and organs. I propose to maintain a laboratory that brings together young scientists who will become leading researchers and developers of imaging methods at the nano scale, with cutting-edge applications in the life sciences. My laboratory will continue to show how imaging can answer important biological questions. My team will continue to develop methods to capture the cell in close to native state and to image cell and tissue slices in 3D down to 1 nanometer resolution. These developments will lead to scientific breakthroughs in the fields of tuberculosis and cancer.
For my laboratory to participate fully in the field of cryo-EM, it was necessary to streamline EM resources in the Netherlands. Therefore, together with Henny Zandbergen I initiated the establishment of the Netherlands Centre for Nanoscopy (NeCEN), and on behalf of 5 Dutch colleagues submitted a grant proposal in 2007. NeCEN was included in the 2008 Roadmap as an essential national facility for EM in life science research. Two top-end Titan Krios cryo-EMs have since come into use at NeCEN in Leiden. These microscopes, representing a total investment of about €18 million, are only useful when the samples have been created and pre-screened using equipment and cryo-TEMs in my own lab. A cryo-FIB/SEM, and a cryo-EM for screening was recently granted by the Province of Limburg and the Dutch Technology Foundation (STW). Use of the facilities at NeCEN will constitute a maximum of 20% of my team’s effort.
In collaboration with Henny Zandbergen, we intend to develop a phase plate located in the rear focal plane of the cryo-TEM to improve contrast. This will allow all phase information to be translated into amplitude information, which will vastly improve the imaging of biological specimens. We have performed tests showing that we can make apertures with the required degree of precision. As we also have a solution to the associated contamination and charging problems, we aim to develop a highly reliable phase plate of the appropriate dimensions. In addition we will be testing new commercial phase plates as they become available.
Since cryo-electron tomography (ET) approaches macromolecular resolution (1-2nm in 3D), it is becoming possible to create maps of individual macromolecular complexes. Most cells, however, are too thick to be imaged intact. Methods such as cryo-sectioning of unperturbed vitreous fully hydrated samples have therefore been introduced for cryo-ET.
As an alternative to vitreous cryo-sectioning, we have recently shown by live-cell light microscopy that migrating white blood cells can naturally migrate within the 1000 nm space of a “nanochamber”. The next step will be to vitrify the cells in this state using novel technology. They are sufficiently thin to be expanded to 200nm using a cryo-FIB/SEM procedures that are yet being developed, making them useful for cryo-ET studies of macromolecular complexes within native mammalian cells without the need for cryo-sectioning. We plan to develop this chamber using silicon-based MEMS technology in collaboration with colleagues at TU Delft and C-flat grids in collaboration with Maastricht Instruments. Two main challenges need to be addressed. First, a chamber must be designed that is even more transparent than the cell, yet still mechanically robust. Second, high throughput methods must be developed to use the chamber efficiently and obtain resolution within the nanometer range. The envisaged device will allow us to study the behavior of intact mammalian cells and their responses to drug-induced changes at the molecular level. The project is ‘high risk’, but if it succeeds it will open up new avenues for nanoscale research on intact mammalian cells.
Type VII secretion system in Mycobacterium tuberculosis; our main biological research question
One way of identifying type VII secretion systems in M. tuberculosis in situ may be through state-of-the-art cryo-correlative light/electron microscopy (cryo-CLEM) studies. We aim to identify this system by imaging GFP-tagged proteins using live-cell microscopy, followed by rapid immobilization in the Vitrobot and cryo-ET. Cryo-ET provides the extreme resolution needed to subsequently construct the detailed 3D image. Currently, the limited resolution (~250 nm) of conventional fluorescence microscopy makes it impossible to unequivocally localize the fluorescent labels to the nanomachines. Approximately 20 GFPs per complex should be detectable by way of correlative cryo-LM and cryo-ET.
Recent advances in optical super-resolution (SR) fluorescence microscopy may remedy this limitation. I recently obtained an STW grant to combine the strengths of cryo-ET and SR-LM by integrating information from both techniques in a single, high-resolution image. To do so, we intend to modify the FEI dedicated cryo-LM holder capable of maintaining the frozen-hydrated EM grid in its vitrified state for use on the SR-LM (FEI TILL Photonics) such that it can work under cryogenic conditions.
Project 1
Type VII secretion system in Mycobacterium tuberculosis
In 2007 our team published an article in Cell showing that after prolonged infection in macrophages and dendritic cells, M. tuberculosis translocated from phago-lysosomes to the cytosol and killed the host cell a few days later, while the BCG vaccine strain failed to translocate. We found that this process was dependent on a gene in the extended RD1 region (ext-RD1). The translocation to the cytosol was unexpected, as it contradicted the prevailing dogma. We then focused on BCG with a knock-in of the entire ext-RD1, as this region is now known to encode the ESX-1 secretion system, which was recently identified by one of our former team member as a novel type VII secretion system (T7SS) (Abdallah AM et al., Nat Rev Microbiol. 2007; see figure below). We found that these bacteria translocate to the cytosol of the host cell 7 days after infection. We concluded that the ESX-1 system (in a BCG background) is sufficient for translocation (Abdallah AM et al., J of Immunol. 2011 and Houben et al., Cell Microbiol. 2012). Therefore we believe that the T7SS is the key to virulence in tuberculosis. Using cryo-EM, we therefore investigated the structure of the mycobacterial capsular layer and showed that high levels of the ESX-1-secreted proteins were present in the capsule (Sani et al. 2010).
We are now using cryo-EM single-particle analysis (SPA), and over the next years will investigate recombinant purified proteins of individual gene products from the T7SS. In addition, we are purifying the entire intact T7SS structure (or mutants thereof) using biochemical methods for 3D reconstruction. Our ultimate aim is to image mycobacteria in vitreous sections in human host cells while translocating. The cryo-SPA, X-ray, and NMR data of the T7SS can then be docked into the images from vitreous sections to construct a macromolecular map of the tubercle bacillus within the host cell. The broader objective is to gain insight into the structure and function of the mechanism for type VII-mediated translocation. This should lay the groundwork for the development of novel antibiotics and better vaccines.
Plunge freezing will be used to obtain a thin vitrified film containing isolated mycobacteria whose outer cell wall has been removed. This will then be subjected to cryo-EM imaging. Purified protein complexes composed of RD1-gene products will be obtained from Stewart Cole in Switzerland, Ravi Ravishankar in India and other scientists worldwide, and subjected to SPA as well as tomographic imaging. This process will give rise to 3D images that can be used as models for host-pathogen interaction. The approaches will be developed with MycobacteriumBCG::RD1 in human cells (adapted to biosafety level 2) as a model for host-pathogen interaction, leading to insights that can be used in the development of new tuberculosis vaccines and drugs. An ERC advanced grant draft for this project is currently being prepared.
Project 2
Cryo-ET with cryo-SR-LM: new tools for imaging nanomachines in cells
Cryo-EM currently provides the ultimate resolution in single-particle analysis (SPA) (<0.5 nm) and in cryo-ET (<2 nm). However, the high information content and low contrast of cryo-EM images often makes it impossible to locate the subcellular structure of interest for cryo-ET. One possible solution may be state-of-the-art correlative light/electron microscopy (CLEM) studies. In these studies, interesting cellular features are identified by imaging GFP-tagged proteins (available for almost all gene products of the genome) using live-cell microscopy, followed by rapid immobilization and cryo-ET. Put simply, cryo-ET provides the extreme resolution needed to construct the detailed 3D image, whereas the proteins areidentified by their genetically encoded fluorescent labels. Currently, the limited resolution (~250 nm) of conventional fluorescence microscopy makes it impossible to unequivocally localize the fluorescent labels to the nanomachines.
To develop this solution, we intend to modify the FEI dedicated cryo-LM holder capable of maintaining the frozen-hydrated EM grid in its vitrified state (recently developed at the Max Planck Institute in Martinsried) for use on the super resolution LM such that it can work under cryogenic conditions. Using this setup, we will then apply PALM principles to obtain optical confocal and later super resolution (SR) cryo-images that identify individual proteins in nanomachines at particular subcellular sites. The preparation will subsequently be transferred to the cryo-EM under cryogenic conditions for ET imaging at sub-nanometer resolution. We will screen the samples with a cryo-TEM (200kV) to select those suitable for cryo-ET at NeCEN. Finally, we will deliver combined image modalities with upwards of 20nm correlation accuracy to identify individual macromolecular machines, which should provide a glimpse into the molecular makeup of the cellular complexes at unprecedented resolution levels.
Successful completion of this task depends on development of several new tools:
- An existing cryo-LM holder will be adapted to fit the SR microscope. This is a challenging task because the potential thermal drift on the stage, caused by liquid nitrogen in the cryo-LM holder, must be kept to an absolute minimum. Further, as immersion oil cannot be used at these temperatures, we will employ a 100 x, 0.9 NA dry objective (Fig. 1).
- We will further develop the MAPS navigation tool, which allows for correlation of the SR images with the cryo-EM images with a relocation precision greater than 50 nm. This requires updating of the existing software to high standards and the introduction of fiducial markers.
This project will benefit from a parallel project to develop novel cryo-ultramicrotomy. In particular, we will collaborate with Diatome to adapt their (room-temperature) oscillating diamond knife to obtain cryo-sections with almost no compression in the preparation.
The project will also benefit from another parallel side project with Maastricht Instruments. Briefly, because intact cells are too thick to be imaged by means of EM without sectioning, we will develop an alternative method called the “double grid approach”. In this device, the cells spread voluntarily to ~200 nm thickness, and can be vitrified with our Vitrobot for ET imaging in a close-to-native state. Maastricht Instruments will integrate an objective lens inside our Vitrobot to observe the migrating cells on a golden EM support grid and manufacture “double film” EM grids. The imaging and screening of these thick preparations by way of cryo-EM requires a ³200 kV.
The developments described here will lead to a better understanding of the positions and states of nanomachines in normal and diseased cells. We will base validation studies of the new instrument on characterization of the new type VII secretion system (see project 1) involved in this translocation. The advances from these studies will greatly benefit not only cryo-ET, but also the SR imaging community. For example, electron microscopists have long known that chemical fixation methods introduce structural artifacts at tens of nm, whereas SR microscopy in STED and PALM mode is routinely performed on formaldehyde-fixed material. This limits the interpretation of high-resolution SR images from a biological perspective. Cryo-SR LM will image proteins in a much closer-to-native preparation. Importantly, the rate of fluorophore bleaching at cryogenic temperatures will be significantly reduced, which means more photons will emit from each fluorophore, resulting in increased resolution. In theory, this effect should far outweigh the lower light-collecting properties of the dry objective.
To achieve the goals laid out in the STW proposal, industry practitioners must develop new tools in collaboration with academic partners. The interest among companies in realizing this aim is evident from their generous offers to contribute. The project will facilitate the development of commercial products that will be in high demand among cryo CLEM labs (academic and industrial) worldwide; namely, a cryogenic chamber, the integration of this chamber with a state-of-the-art SR microscope, and tools to facilitate rapid relocalization of the preparation from the SR setup to the EM.
Project 3
Nanofluidic chamber for cryo-ET
Cryo-ET of vitreous sections is an emerging technique that produces high-resolution (2–4 nm) structures of macromolecular complexes in frozen-hydrated state within their native environment. High-pressure frozen cells are sectioned into long ribbons 50nm thick, and viewed using cryo-EM. This approach is technically demanding, and it is difficult to ascertain whether the macromolecular complexes are disrupted by the freezing and sectioning processes. While it has been shown that whole bacterial cells can be visualized without sectioning (Kühner et al. Science 2009), the electron scattering prevents high-resolution imaging for organic samples thicker than 200 nm, which renders mammalian cells (at several microns) too thick. As an alternative to vitreous cryo-sectioning, we recently showed by way of live-cell light microscopy that migrating white blood cells (DCs, T-cells, etc.) can naturally migrate into a 1000 nm thick “nanochamber”. Using nano technology, we now intend to develop a method to vitrify the cells in this state. They are then sufficiently thin to be subjected to a cryo-FIB/SEM procedure making them 200nm, and thus useful for cryo-ET studies of macromolecular complexes within native mammalian cells without the need for cryo-sectioning.
My lab will develop this chamber with silicon-based microelectromechanical systems (MEMS) technology in collaboration with colleagues at TU Delft. Two main challenges need to be addressed. First, a chamber must be designed that is even more transparent than the cell, yet still mechanically robust. This chamber should fit seamlessly with the existing high-tech equipment: cryo-EMs, cryo-fluorescent microscopes, and cell preparation tools. Second, high throughput methods must be developed to use the chamber efficiently and obtain resolution within the nanometer range. The envisaged device will allow us to study the behavior of intact mammalian cells and their responses to drug-induced changes at the molecular level. The project is ‘high risk’, but could open up new avenues for nanoscale research on intact mammalian cells. If successful, we intend to investigate human dendritic cells infected with M. marinum as well as the macromolecular interaction of T7SS with the phagolysosomal membrane.
Project 4
Effects of linezolid on ribosomes of M. tuberculosis: a structure-function study
At present, drug-induced changes of macromolecular targets cannot be observed within the native cellular environment. Ideally, a technique must be developed that allows for the observation of high-resolution macromolecular structure changes within this environment. One promising antibiotic for the treatment of multi-drug resistant (MDR) tuberculosis is linezolid.
Linezolid acts by inhibiting bacterial protein synthesis. The available X-ray crystal structures of linezolid in complex with 50S ribosome of Haloarcula marismortui (an archaeon) suggest that linezolid may inhibit the formation of the ribosomal initiation complex (Joseph et al., J Med Chem. 2008). As yet, however, the mechanism of action of linezolid on M. tuberculosis has not been definitively identified. This project focuses on the effect of linezolid on ribosomes isolated from mycobacteria, with a view to answering the following questions:
- Does linezolid prevent the formation of functional mycobacterial ribosome initiating complexes in vitro?
- Does linezolid have the same mechanism of action in vivo (within the context of the entire bacterial cell)?
We will evaluate the quality of the ribosome preparation by means of cryo-EM. Using the same technique, we will also study the effect of linezolid on the formation of mycobacterial 70S ribosomes and determine the optimal concentration for inhibiting complex formation. Subsequently, we will investigate the effect of linezolid on in vitro purified mycobacterial ribosomes and on M. tuberculosis cells to determine the minimal inhibitory concentration of antibiotic that has a bactericidal effect on our strains. To this end, we will use fluorescent microscopy to observe bacterial cell death with Live/DEAD BAC/Light assay. Finally, we will prepare M. tuberculosis treated with linezolid for cryo-EM of vitreous sections using high-pressure freezing (HPF) and vitreous cryo-sectioning techniques (Pierson et al. J Structural Biology 2011) or cryo-FIB/SEM lamellae to identify the mechanism of action of drugs on ribosomes inside entire bacterial cells.
This project is intended as a prototype for structural characterization of mycobacterial ribosome. It will provide the research team with scripts and protocols for SP-cryo EM designed to tackle specific issues that may arise during ribosome reconstruction.
Project 5
New technology improvement for the cryo-EM: phase plate
If a specimen merely changes the phase of the electron wave (i.e. the specimen is a phase object), provided the electron microscope is at zero focus and there are no aberrations, there will be nothing to observe. That information was obtained from biological specimens in the past was partly due to the poor optical performance of the TEMs, and partly due to the use of a relatively large defocus. However, a significant amount of information is then lost. Contemporary electron microscopes can be equipped with an aberration corrector, and at zero focus on a phase object virtually nothing will be observed. Yet switching to off-focus also results in a loss of information. Thus, a phase plate – similar to the Zernike phase plate in light microscopy – would clearly be desirable. This phase plate should effect a phase shift of pi/2 on the central beam compared to the diffracted beams, and should not block the relevant electrons. In biology, all information beyond 0.01 Å – apart from the phase-shifted central beam – should be passed up to 1 Å through the phase plate. The phase plate must be located in the rear focal plane of the TEM. It will allow all phase information to be translated into amplitude information, which will vastly improve the imaging of biological specimens.
Several research groups have experimented with phase plates. Two types of phase plates can be identified: a) a thin sheet of C, for instance, can be placed such that all diffracted beams have a phase shift of pi/2; or b) the central beam is led through a small tube that effects a phase shift on the central beam. The second approach is the more appropriate. However, although such phase plates have been used successfully for over 5 years, no commercial product is yet available. This can be attributed to the difficulties involved in developing them. Not only must they be extremely small (with ~50 nm precision); the issues of contamination and charging also pose problems. In collaboration with Henny Zandbergen and his team at the Kavli Nanoscience Institute (TU Delft), we have performed tests showing that we can make apertures with the required degree of precision. As we also have solution to the contamination and charging problems, we aim to develop a reliable phase plate of the appropriate dimensions.
Project 6
Deciphering inter cellular signalling in schizophrenia
Start date 2014
Chronic mental illnesses (CMI) like schizophrenia or the recurrent affective disorders are among the most debilitating (prevalence 1-10%) and costly diseases in the Western world. Research on brain diseases is a focus in FP7. In fact, direct healthcare costs of the two disease categories that IN-SENS is directly addressing, the psychotic and affective disorders cost four times as much as dementia and other neurodegenerative disorders. This is mainly due to the early onset of CMI in adolescence which leads to in most cases lifelong impairments and occupational disability. Yet, even though CMI have dramatic impact on individual patients, their relatives, and society, our biological understanding and, accordingly, our options for novel strategies of efficient treatment or cure of CMI are stalling.
The last decade has brought breakthroughs in the genetics of CMI, like the discovery of the disrupted-in-schizophrenia 1 (DISC1) gene (by a member of this consortium), and others. The identification of these candidate genes now allows analysis of the INter- and intracellular Signalling INSchizophrenia (hence the project acronym IN-SENS). A European perspective is necessary since in most EU countries the infrastructure for the necessary interdisciplinary research is not met on national levels. In Maastricht we will be doing cryo-immunogold EM to localize DISC1 in the healthy and diseased brain.
Project 7
Human in vitro tissue and single cell approaches to model tuberculosis
Start date 2014
An urgent task of today’s medical research is to find new ways to treat infections caused by Mycobacterium tuberculosis. There are numerous reports on multidrug-resistant strains of this pathogen, which causes tuberculosis (TB). Most experimental studies of TB rely on animal experiments and there are no existing alternative models such as in vitro tissue models, with the exception of infected single cell cultures. Replacing existing animal models with human in vitro systems is also important because of the questioned validity of these models for TB. The aim of the presented project is to further develop two novel human in vitro models for TB, validate them against tissues from TB patients and to use them instead of animals for testing anti-TB combination regimens based on existing drugs. The models are based on human primary cells and cell lines and the investigation of these models as substitutes for animal models for preclinical drug testing.
The first model is based on macrophages obtained from human blood that are infected with virulent M. tuberculosis. Similar models have been described, but the unique feature of the presented model that the model displays is that under the right conditions, it can mimic clinical latent TB. Understanding latent TB is of importance, since the latent infection remaining in patients on TB treatment is very tolerant towards antibiotics and is causative of multidrug resistance. The so far confirmed results include absence of net growth of the bacteria inside the macrophages over two weeks, alteration of characteristics of the bacteria identical to observations in other studies, suppression of immune activation of the infected cells, tolerance towards antibiotics and most importantly, regrowth of the bacteria during immunosuppression, which is a well-known clinical problem and has so far only been modeled in animals. The second model is based on the establishment of co-cultures of human cell lines and primary immune cells on a filter with a collagen matrix. Human blood monocyte-derived macrophages infected with M. tuberculosis are introduced into the system. The tissues are exposed to air on the apical side, causing the epithelial cells to secrete mucus, further mimicking the microenvironment in the lung. This unique TB model will be characterized within the project. During the present project, the models will be compared with and validated against human TB-infected tissues on an ultrastructural level using advanced electron microscopy (Maastricht University). The proposed project is a translational academic project linking experimental research to clinical testing and including Dutch and Swedish research groups. The models will be used for screening combinations of approved drugs together with TB-antibiotics and for achieving proof-of-principle of adjuvant treatments for a more effective TB treatment. Together, the two models may prove to serve as good substitutes for animal models for TB. If the project is successful, the models can be further developed to mimic different kinds of bacterial and viral infections.
Project 8
Collaboration with Hans Clevers:
Project proposal in preparation
NC3Rs – National Centre for the Replacement, Refinement and Reduction of Animals in Research
Advancing the development and application of non-animal technologies - NEW COMPETITION FOR FEASIBILITY STUDY FUNDING
Publications
1.
The use of a central beam stop for contrast enhancement in TEM imaging.
Zhang C, Xu Q, Peters PJ, Zandbergen H.
Ultramicroscopy. 2013 Nov;134:200-6. doi: 10.1016/j.ultramic.2013.05.019. Epub 2013 Jun 5.
2.
Stange DE, Koo BK, Huch M, Sibbel G, Basak O, Lyubimova A, Kujala P, Bartfeld S, Koster J, Geahlen JH, Peters PJ, van Es JH, van de Wetering M, Mills JC, Clevers H.
Cell. 2013 Oct 10;155(2):357-68. doi: 10.1016/j.cell.2013.09.008.
3.
Godsave SF, Wille H, Pierson J, Prusiner SB, Peters PJ.
Neurobiol Aging. 2013 Jun;34(6):1621-31. doi: 10.1016/j.neurobiolaging.2012.12.015.
4.
Lgr5(+ve) stem/progenitor cells contribute to nephron formation during kidney development.
Barker N, Rookmaaker MB, Kujala P, Ng A, Leushacke M, Snippert H, van de Wetering M, Tan S, Van Es JH, Huch M, Poulsom R, Verhaar MC, Peters PJ, Clevers H.
Cell Rep. 2012 Sep 27;2(3):540-52. doi: 10.1016/j.celrep.2012.08.018. Epub 2012 Sep 20.
5.
de Lau W, Kujala P, Schneeberger K, Middendorp S, Li VS, Barker N, Martens A, Hofhuis F, DeKoter RP, Peters PJ, Nieuwenhuis E, Clevers H.
Mol Cell Biol. 2012 Sep;32(18):3639-47. doi: 10.1128/MCB.00434-12. Epub 2012 Jul 9.
6.
ESX-1-mediated translocation to the cytosol controls virulence of mycobacteria.
Houben D, Demangel C, van Ingen J, Perez J, Baldeón L, Abdallah AM, Caleechurn L, Bottai D, van Zon M, de Punder K, van der Laan T, Kant A, Bossers-de Vries R, Willemsen P, Bitter W, van Soolingen D, Brosch R, van der Wel N, Peters PJ.
Cell Microbiol. 2012 Aug;14(8):1287-98. doi: 10.1111/j.1462-5822.2012.01799.x. Epub 2012 May 8.
7.
Prion uptake in the gut: identification of the first uptake and replication sites.
Kujala P, Raymond CR, Romeijn M, Godsave SF, van Kasteren SI, Wille H, Prusiner SB, Mabbott NA, Peters PJ.
PLoS Pathog. 2011 Dec;7(12):e1002449. doi: 10.1371/journal.ppat.1002449. Epub 2011 Dec 22.
8.
Abdallah AM, Bestebroer J, Savage ND, de Punder K, van Zon M, Wilson L, Korbee CJ, van der Sar AM, Ottenhoff TH, van der Wel NN, Bitter W, Peters PJ.
J Immunol. 2011 Nov 1;187(9):4744-53. doi: 10.4049/jimmunol.1101457. Epub 2011 Sep 28.
9.
Saurí A, Oreshkova N, Soprova Z, Jong WS, Sani M, Peters PJ, Luirink J, van Ulsen P.
J Mol Biol. 2011 Sep 30;412(4):553-67. doi: 10.1016/j.jmb.2011.07.035. Epub 2011 Jul 23.
10.
Lgr5 homologues associate with Wnt receptors and mediate R-spondin signalling.
de Lau W, Barker N, Low TY, Koo BK, Li VS, Teunissen H, Kujala P, Haegebarth A, Peters PJ, van de Wetering M, Stange DE, van Es JE, Guardavaccaro D, Schasfoort RB, Mohri Y, Nishimori K, Mohammed S, Heck AJ, Clevers H.
Nature. 2011 Jul 4;476(7360):293-7. doi: 10.1038/nature10337.
11.
Bos E, SantAnna C, Gnaegi H, Pinto RF, Ravelli RB, Koster AJ, de Souza W, Peters PJ.
J Struct Biol. 2011 Jul;175(1):62-72. doi: 10.1016/j.jsb.2011.03.022. Epub 2011 Apr 5.
12.
Daleke MH, Cascioferro A, de Punder K, Ummels R, Abdallah AM, van der Wel N, Peters PJ, Luirink J, Manganelli R, Bitter W.
J Biol Chem. 2011 May 27;286(21):19024-34. doi: 10.1074/jbc.M110.204966. Epub 2011 Apr 6.
13.
Pierson J, Ziese U, Sani M, Peters PJ.
J Struct Biol. 2011 Feb;173(2):345-9. doi: 10.1016/j.jsb.2010.09.017. Epub 2010 Sep 21.
14.
Perspectives on electron cryo-tomography of vitreous cryo-sections.
Pierson J, Vos M, McIntosh JR, Peters PJ.
J Electron Microsc (Tokyo). 2011;60 Suppl 1:S93-100. doi: 10.1093/jmicro/dfr014. Review.
15.
Sani M, Houben EN, Geurtsen J, Pierson J, de Punder K, van Zon M, Wever B, Piersma SR, Jiménez CR, Daffé M, Appelmelk BJ, Bitter W, van der Wel N, Peters PJ.
PLoS Pathog. 2010 Mar 5;6(3):e1000794. doi: 10.1371/journal.ppat.1000794.
16.
Pierson J, Fernández JJ, Bos E, Amini S, Gnaegi H, Vos M, Bel B, Adolfsen F, Carrascosa JL, Peters PJ.
J Struct Biol. 2010 Feb;169(2):219-25. doi: 10.1016/j.jsb.2009.10.001. Epub 2009 Oct 12.
17.
Lgr5(+ve) stem cells drive self-renewal in the stomach and build long-lived gastric units in vitro.
Barker N, Huch M, Kujala P, van de Wetering M, Snippert HJ, van Es JH, Sato T, Stange DE, Begthel H, van den Born M, Danenberg E, van den Brink S, Korving J, Abo A, Peters PJ, Wright N, Poulsom R, Clevers H.
Cell Stem Cell. 2010 Jan 8;6(1):25-36. doi: 10.1016/j.stem.2009.11.013.
18.
How do mycobacteria activate CD8+ T cells?
Weerdenburg EM, Peters PJ, van der Wel NN.
Trends Microbiol. 2010 Jan;18(1):1-10. doi: 10.1016/j.tim.2009.10.004. Epub 2009 Dec 3.
19.
Gregorieff A, Stange DE, Kujala P, Begthel H, van den Born M, Korving J, Peters PJ, Clevers H.
Gastroenterology. 2009 Oct;137(4):1333-45.e1-3. doi: 10.1053/j.gastro.2009.06.044. Epub 2009 Jun 21.
20.
Toward visualization of nanomachines in their native cellular environment.
Pierson J, Sani M, Tomova C, Godsave S, Peters PJ.
Histochem Cell Biol. 2009 Sep;132(3):253-62. doi: 10.1007/s00418-009-0622-0. Epub 2009 Aug 1. Review.
21.
Acid sphingomyelinase is a key regulator of cytotoxic granule secretion by primary T lymphocytes.
Herz J, Pardo J, Kashkar H, Schramm M, Kuzmenkina E, Bos E, Wiegmann K, Wallich R, Peters PJ, Herzig S, Schmelzer E, Krönke M, Simon MM, Utermöhlen O.
Nature Immunol. 2009 Jul;10(7):761-8. doi: 10.1038/ni.1757. Epub 2009 Jun 14.
22.
Single Lgr5 stem cells build crypt-villus structures in vitro without a mesenchymal niche.
Sato T, Vries RG, Snippert HJ, van de Wetering M, Barker N, Stange DE, van Es JH, Abo A, Kujala P, Peters PJ, Clevers H.
Nature. 2009 May 14;459(7244):262-5. doi: 10.1038/nature07935. Epub 2009 Mar 29.
23.
Transcription factor achaete scute-like 2 controls intestinal stem cell fate.
van der Flier LG, van Gijn ME, Hatzis P, Kujala P, Haegebarth A, Stange DE, Begthel H, van den Born M, Guryev V, Oving I, van Es JH, Barker N, Peters PJ, van de Wetering M, Clevers H.
Cell. 2009 Mar 6;136(5):903-12. doi: 10.1016/j.cell.2009.01.031.
24.
A role for the host coatomer and KDEL receptor in early vaccinia biogenesis.
Zhang L, Lee SY, Beznoussenko GV, Peters PJ, Yang JS, Gilbert HY, Brass AL, Elledge SJ, Isaacs SN, Moss B, Mironov A, Hsu VW.
Proc Natl Acad Sci U S A. 2009 Jan 6;106(1):163-8. doi: 10.1073/pnas.0811631106. Epub 2008 Dec 24.
25.
Cryo-immunogold electron microscopy for prions: toward identification of a conversion site.
Godsave SF, Wille H, Kujala P, Latawiec D, DeArmond SJ, Serban A, Prusiner SB, Peters PJ.
J Neurosci. 2008 Nov 19;28(47):12489-99. doi: 10.1523/JNEUROSCI.4474-08.2008.
26.
Hava DL, van der Wel N, Cohen N, Dascher CC, Houben D, León L, Agarwal S, Sugita M, van Zon M, Kent SC, Shams H, Peters PJ, Brenner MB.
Proc Natl Acad Sci U S A. 2008 Aug 12;105(32):11281-6. doi: 10.1073/pnas.0804681105. Epub 2008 Aug 6.
27.
Verbrugghe P, Kujala P, Waelput W, Peters PJ, Cuvelier CA.
Histochem Cell Biol. 2008 Mar;129(3):311-20. Epub 2007 Dec 21.
28.
Immunogold labeling of thawed cryosections.
Peters PJ, Pierson J.
Methods Cell Biol. 2008;88:131-49. doi: 10.1016/S0091-679X(08)00408-1. Review. No abstract available.
29.
Identification of stem cells in small intestine and colon by marker gene Lgr5.
Barker N, van Es JH, Kuipers J, Kujala P, van den Born M, Cozijnsen M, Haegebarth A, Korving J, Begthel H, Peters PJ, Clevers H.
Nature. 2007 Oct 25;449(7165):1003-7. Epub 2007 Oct 14.
30.
Knöll R, Postel R, Wang J, Krätzner R, Hennecke G, Vacaru AM, Vakeel P, Schubert C, Murthy K, Rana BK, Kube D, Knöll G, Schäfer K, Hayashi T, Holm T, Kimura A, Schork N, Toliat MR, Nürnberg P, Schultheiss HP, Schaper W, Schaper J, Bos E, Den Hertog J, van Eeden FJ, Peters PJ, Hasenfuss G, Chien KR, Bakkers J.
Circulation. 2007 Jul 31;116(5):515-25. Epub 2007 Jul 23.
31.
An ACAP1-containing clathrin coat complex for endocytic recycling.
Li J, Peters PJ, Bai M, Dai J, Bos E, Kirchhausen T, Kandror KV, Hsu VW.
J Cell Biol. 2007 Jul 30;178(3):453-64.
32.
M. tuberculosis and M. leprae translocate from the phagolysosome to the cytosol in myeloid cells.
van der Wel N, Hava D, Houben D, Fluitsma D, van Zon M, Pierson J, Brenner M, Peters PJ.
Cell. 2007 Jun 29;129(7):1287-98.
33.
Electron tomography of bacterial chemotaxis receptor assemblies.
Zhang P, Weis RM, Peters PJ, Subramaniam S.
Methods Cell Biol. 2007;79:373-84. Review. No abstract available.
34.
Klingenstein R, Löber S, Kujala P, Godsave S, Leliveld SR, Gmeiner P, Peters PJ, Korth C.
J Neurochem. 2006 Aug;98(3):748-59. Epub 2006 Jun 2. Erratum in: J Neurochem. 2006 Sep;98(5):1696.
35.
Emerging pharmacotherapies for Creutzfeldt-Jakob disease.
Korth C, Peters PJ.
Arch Neurol. 2006 Apr;63(4):497-501. Review.
36.
Cryo-immunogold electron microscopy.
Peters PJ, Bos E, Griekspoor A.
Curr Protoc Cell Biol. 2006 Apr;Chapter 4:Unit 4.7. doi: 10.1002/0471143030.cb0407s30.
37.
Quantitative and dynamic assessment of the contribution of the ER to phagosome formation.
Touret N, Paroutis P, Terebiznik M, Harrison RE, Trombetta S, Pypaert M, Chow A, Jiang A, Shaw J, Yip C, Moore HP, van der Wel N, Houben D, Peters PJ, de Chastellier C, Mellman I, Grinstein S.
Cell. 2005 Oct 7;123(1):157-70.
38.
In vivo control of endosomal architecture by class II-associated invariant chain and cathepsin S.
Boes M, van der Wel N, Peperzak V, Kim YM, Peters PJ, Ploegh H.
Eur J Immunol. 2005 Sep;35(9):2552-62.
39.
van der Wel NN, Fluitsma DM, Dascher CC, Brenner MB, Peters PJ.
Curr Opin Microbiol. 2005 Jun;8(3):323-30. Review.
40.
ACAP1 promotes endocytic recycling by recognizing recycling sorting signals.
Dai J, Li J, Bos E, Porcionatto M, Premont RT, Bourgoin S, Peters PJ, Hsu VW.
Dev Cell. 2004 Nov;7(5):771-6.
41.
Nolte-'t Hoen EN, Wagenaar-Hilbers JP, Peters PJ, Gadella BM, van Eden W, Wauben MH.
Eur J Immunol. 2004 Nov;34(11):3115-25.
42.
Zhang P, Bos E, Heymann J, Gnaegi H, Kessel M, Peters PJ, Subramaniam S.
J Microsc. 2004 Oct;216(Pt 1):76-83.
43.
Lefman J, Zhang P, Hirai T, Weis RM, Juliani J, Bliss D, Kessel M, Bos E, Peters PJ, Subramaniam S.
J Bacteriol. 2004 Aug;186(15):5052-61.
44.
Griparic L, van der Wel NN, Orozco IJ, Peters PJ, van der Bliek AM.
J Biol Chem. 2004 Apr 30;279(18):18792-8. Epub 2004 Feb 16.
45.
Complete polarization of single intestinal epithelial cells upon activation of LKB1 by STRAD.
Baas AF, Kuipers J, van der Wel NN, Batlle E, Koerten HK, Peters PJ, Clevers HC.
Cell. 2004 Feb 6;116(3):457-66.
46.
Catalfamo M, Karpova T, McNally J, Costes SV, Lockett SJ, Bos E, Peters PJ, Henkart PA.
Immunity. 2004 Feb;20(2):219-30.
47.
The transferrin receptor: a potential molecular imaging marker for human cancer.
Högemann-Savellano D, Bos E, Blondet C, Sato F, Abe T, Josephson L, Weissleder R, Gaudet J, Sgroi D, Peters PJ, Basilion JP.
Neoplasia. 2003 Nov-Dec;5(6):495-506.
48.
Lysosomal localization of murine CD1d mediated by AP-3 is necessary for NK T cell development.
Cernadas M, Sugita M, van der Wel N, Cao X, Gumperz JE, Maltsev S, Besra GS, Behar SM, Peters PJ, Brenner MB.
J Immunol. 2003 Oct 15;171(8):4149-55.
49.
Trafficking of prion proteins through a caveolae-mediated endosomal pathway.
Peters PJ, Mironov A Jr, Peretz D, van Donselaar E, Leclerc E, Erpel S, DeArmond SJ, Burton DR, Williamson RA, Vey M, Prusiner SB.
J Cell Biol. 2003 Aug 18;162(4):703-17.
50.
Cytosolic prion protein in neurons.
Mironov A Jr, Latawiec D, Wille H, Bouzamondo-Bernstein E, Legname G, Williamson RA, Burton D, DeArmond SJ, Prusiner SB, Peters PJ.
J Neurosci. 2003 Aug 6;23(18):7183-93.
51.
van der Wel NN, Sugita M, Fluitsma DM, Cao X, Schreibelt G, Brenner MB, Peters PJ.
Mol Biol Cell. 2003 Aug;14(8):3378-88. Epub 2003 Jun 13.
52.
Weis RM, Hirai T, Chalah A, Kessel M, Peters PJ, Subramaniam S.
J Bacteriol. 2003 Jun;185(12):3636-43.
53.
Morphology and dynamics of clathrin/GGA1-coated carriers budding from the trans-Golgi network.
Puertollano R, van der Wel NN, Greene LE, Eisenberg E, Peters PJ, Bonifacino JS.
Mol Biol Cell. 2003 Apr;14(4):1545-57.
54.
Cao X, Sugita M, Van Der Wel N, Lai J, Rogers RA, Peters PJ, Brenner MB.
J Immunol. 2002 Nov 1;169(9):4770-7.
55.
Arfaptin 2 regulates the aggregation of mutant huntingtin protein.
Peters PJ, Ning K, Palacios F, Boshans RL, Kazantsev A, Thompson LM, Woodman B, Bates GP, D'Souza-Schorey C.
Nature Cell Biol. 2002 Mar;4(3):240-5.
56.
Characterization of coated vesicles that participate in endocytic recycling.
Peters PJ, Gao M, Gaschet J, Ambach A, van Donselaar E, Traverse JF, Bos E, Wolffe EJ, Hsu VW.
Traffic. 2001 Dec;2(12):885-95.
57.
The p41 isoform of invariant chain is a chaperone for cathepsin L.
Lennon-Duménil AM, Roberts RA, Valentijn K, Driessen C, Overkleeft HS, Erickson A, Peters PJ, Bikoff E, Ploegh HL, Wolf Bryant P.
EMBO J. 2001 Aug 1;20(15):4055-64.
58.
Peters PJ, Hunziker W.
Methods Enzymol. 2001;329:210-25. No abstract available.
59.
Ameen NA, van Donselaar E, Posthuma G, de Jonge H, McLaughlin G, Geuze HJ, Marino C, Peters PJ.
Histochem Cell Biol. 2000 Sep;114(3):219-28.
60.
CD1c molecules broadly survey the endocytic system.
Sugita M, van Der Wel N, Rogers RA, Peters PJ, Brenner MB.
Proc Natl Acad Sci U S A. 2000 Jul 18;97(15):8445-50.
61.
Sugita M, Peters PJ, Brenner MB.
Traffic. 2000 Apr;1(4):295-300. Review.
62.
Self-recognition of CD1 by gamma/delta T cells: implications for innate immunity.
Spada FM, Grant EP, Peters PJ, Sugita M, Melián A, Leslie DS, Lee HK, van Donselaar E, Hanson DA, Krensky AM, Majdic O, Porcelli SA, Morita CT, Brenner MB.
J Exp Med. 2000 Mar 20;191(6):937-48.
63.
Separate pathways for antigen presentation by CD1 molecules.
Sugita M, Grant EP, van Donselaar E, Hsu VW, Rogers RA, Peters PJ, Brenner MB.
Immunity. 1999 Dec;11(6):743-52.
64.
Franco M, Peters PJ, Boretto J, van Donselaar E, Neri A, D'Souza-Schorey C, Chavrier P.
EMBO J. 1999 Mar 15;18(6):1480-91.
65.
Hochstenbach F, Klis FM, van den Ende H, van Donselaar E, Peters PJ, Klausner RD.
Proc Natl Acad Sci U S A. 1998 Aug 4;95(16):9161-6.
66.
Depletion of epithelial stem-cell compartments in the small intestine of mice lacking Tcf-4.
Korinek V, Barker N, Moerer P, van Donselaar E, Huls G, Peters PJ, Clevers H.
Nature Genet. 1998 Aug;19(4):379-83.
67.
Hunziker W, Peters PJ.
J Biol Chem. 1998 Jun 19;273(25):15734-41.
68.
CD1--a new paradigm for antigen presentation and T cell activation.
Sugita M, Moody DB, Jackman RM, Grant EP, Rosat JP, Behar SM, Peters PJ, Porcelli SA, Brenner MB.
Clin Immunol Immunopathol. 1998 Apr;87(1):8-14. Review.
69.
Jackman RM, Stenger S, Lee A, Moody DB, Rogers RA, Niazi KR, Sugita M, Modlin RL, Peters PJ, Porcelli SA.
Immunity. 1998 Mar;8(3):341-51.
70.
Aoe T, Lee AJ, van Donselaar E, Peters PJ, Hsu VW.
Proc Natl Acad Sci U S A. 1998 Feb 17;95(4):1624-9.
71.
D'Souza-Schorey C, van Donselaar E, Hsu VW, Yang C, Stahl PD, Peters PJ.
J Cell Biol. 1998 Feb 9;140(3):603-16.
72.
de Lau WB, Kuipers J, Peters PJ, Lokhorst HM, Clevers H, Bast BJ.
Leuk Res. 1998 Feb;22(2):163-73.
73.
Current views in intracellular transport: insights from studies in immunology.
Hsu VW, Peters PJ.
Adv Immunol. 1998;70:369-415. Review. No abstract available.
74.
Aoe T, Cukierman E, Lee A, Cassel D, Peters PJ, Hsu VW.
EMBO J. 1997 Dec 15;16(24):7305-16.
75.
The CD1-restricted T-cell response to mycobacteria.
Moody DB, Sugita M, Peters PJ, Brenner MB, Porcelli SA.
Res Immunol. 1996 Oct-Dec;147(8-9):550-9. Review. No abstract available.
76.
Cytoplasmic tail-dependent localization of CD1b antigen-presenting molecules to MIICs.
Sugita M, Jackman RM, van Donselaar E, Behar SM, Rogers RA, Peters PJ, Brenner MB, Porcelli SA.
Science. 1996 Jul 19;273(5273):349-52.
77.
Wolffe EJ, Moore DM, Peters PJ, Moss B.
J Virol. 1996 May;70(5):2797-808.
78.
Voorhees P, Deignan E, van Donselaar E, Humphrey J, Marks MS, Peters PJ, Bonifacino JS.
EMBO J. 1995 Oct 16;14(20):4961-75.
79.
Marks MS, Roche PA, van Donselaar E, Woodruff L, Peters PJ, Bonifacino JS.
J Cell Biol. 1995 Oct;131(2):351-69.
80.
Peters PJ, Raposo G, Neefjes JJ, Oorschot V, Leijendekker RL, Geuze HJ, Ploegh HL.
J Exp Med. 1995 Aug 1;182(2):325-34.
81.
Peters PJ, Hsu VW, Ooi CE, Finazzi D, Teal SB, Oorschot V, Donaldson JG, Klausner RD.
J Cell Biol. 1995 Mar;128(6):1003-17.
82.
The ARF GTPases: defining roles in membrane traffic and organelle structure.
Donaldson JG, Radhakrishna H, Peters PJ.
Cold Spring Harb Symp Quant Biol. 1995;60:229-34. Review. No abstract available.
83.
Bosshart H, Humphrey J, Deignan E, Davidson J, Drazba J, Yuan L, Oorschot V, Peters PJ, Bonifacino JS.
J Cell Biol. 1994 Sep;126(5):1157-72.
84.
An activating mutation in ARF1 stabilizes coatomer binding to Golgi membranes.
Teal SB, Hsu VW, Peters PJ, Klausner RD, Donaldson JG.
J Biol Chem. 1994 Feb 4;269(5):3135-8.
85.
Humphrey JS, Peters PJ, Yuan LC, Bonifacino JS.
J Cell Biol. 1993 Mar;120(5):1123-35.
86.
Peptide-induced stabilization and intracellular localization of empty HLA class I complexes.
Baas EJ, van Santen HM, Kleijmeer MJ, Geuze HJ, Peters PJ, Ploegh HL.
J Exp Med. 1992 Jul 1;176(1):147-56.
87.
Van Eijkeren MA, Peters PJ, Geuze HJ.
Eur J Immunol. 1991 Dec;21(12):3049-52.
88.
Parmentier HK, Van Wichen DF, Peters PJ, Tschopp J, De Weger RA, Schuurman HJ.
AIDS. 1991 Jun;5(6):778-80. No abstract available.
89.
Cytotoxic T lymphocyte granules are secretory lysosomes, containing both perforin and granzymes.
Peters PJ, Borst J, Oorschot V, Fukuda M, Krähenbühl O, Tschopp J, Slot JW, Geuze HJ.
J Exp Med. 1991 May 1;173(5):1099-109.
90.
Peters PJ, Neefjes JJ, Oorschot V, Ploegh HL, Geuze HJ.
Nature. 1991 Feb 21;349(6311):669-76.
91.
Metzelaar MJ, Wijngaard PL, Peters PJ, Sixma JJ, Nieuwenhuis HK, Clevers HC.
J Biol Chem. 1991 Feb 15;266(5):3239-45.
92.
Masson D, Peters PJ, Geuze HJ, Borst J, Tschopp J.
Biochemistry. 1990 Dec 25;29(51):11229-35.
93.
The biosynthetic pathway of MHC class II but not class I molecules intersects the endocytic route.
Neefjes JJ, Stollorz V, Peters PJ, Geuze HJ, Ploegh HL.
Cell. 1990 Apr 6;61(1):171-83.
94.
Teunissen MB, Wormmeester J, Krieg SR, Peters PJ, Vogels IM, Kapsenberg ML, Bos JD.
J Invest Dermatol. 1990 Feb;94(2):166-73.
95.
A new model for lethal hit delivery by cytotoxic T lymphocytes.
Peters PJ, Geuze HJ, van der Donk HA, Borst J.
Immunol Today. 1990 Jan;11(1):28-32. Review.
96.
Stam NJ, Vroom TM, Peters PJ, Pastoors EB, Ploegh HL.
Int Immunol. 1990;2(2):113-25.
97.
Eradication of adenovirus E1-induced tumors by E1A-specific cytotoxic T lymphocytes.
Kast WM, Offringa R, Peters PJ, Voordouw AC, Meloen RH, van der Eb AJ, Melief CJ.
Cell. 1989 Nov 17;59(4):603-14.
98.
Peters PJ, Geuze HJ, Van der Donk HA, Slot JW, Griffith JM, Stam NJ, Clevers HC, Borst J.
Eur J Immunol. 1989 Aug;19(8):1469-75.
99.
Immunosurveillance of virus-induced tumors.
Melief CJ, Vasmel WL, Offringa R, Sijts EJ, Matthews EA, Peters PJ, Meloen RH, van der Eb AJ, Kast WM.
Cold Spring Harb Symp Quant Biol. 1989;54 Pt 1:597-603. Review. No abstract available.
100.
Borst J, van Dongen JJ, Bolhuis RL, Peters PJ, Hafler DA, de Vries E, van de Griend RJ.
J Exp Med. 1988 May 1;167(5):1625-44.
101.
Peters JP, Rademakers LH, de Boer RJ, Van Unnik JA.
J Pathol. 1987 Nov;153(3):233-44.
102.
Lokhorst HM, Boom SE, Bast BJ, Peters PJ, Tedder TF, Gerdes J, Petersen E, Ballieux RE.
J Clin Invest. 1987 May;79(5):1401-11.
103.
Kluin PM, de Weger RA, Schuurman HJ, Peters PP, Spies PI, Bast BJ, van Unnik JA, de Gast GC.
Scand J Haematol. 1985 Oct;35(4):399-407.
104.
Rademakers LH, Peters JP, Roelofs JM, van Unnik JA.
Adv Exp Med Biol. 1985;186:993-8. No abstract available.
105.
Morphology and enzyme histochemistry of isolated human follicular dendritic cells.
Rademakers LH, Peters JP, Go DM, de Weger RA, van Unnik JA.
Cell Biol Int Rep. 1984 Mar;8(3):185. No abstract available.
106.
Peters JP, Rademakers LH, Roelofs JM, de Jong D, van Unnik JA.
Virchows Arch B Cell Pathol Incl Mol Pathol. 1984;46(3):215-28.
107.
Rademakers LH, Peters JP, van Unnik JA.
Virchows Arch B Cell Pathol Incl Mol Pathol. 1983;44(1):85-98.
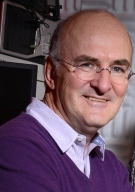
Peter J. Peters (J.P.J.)
Professor of Nanobiology